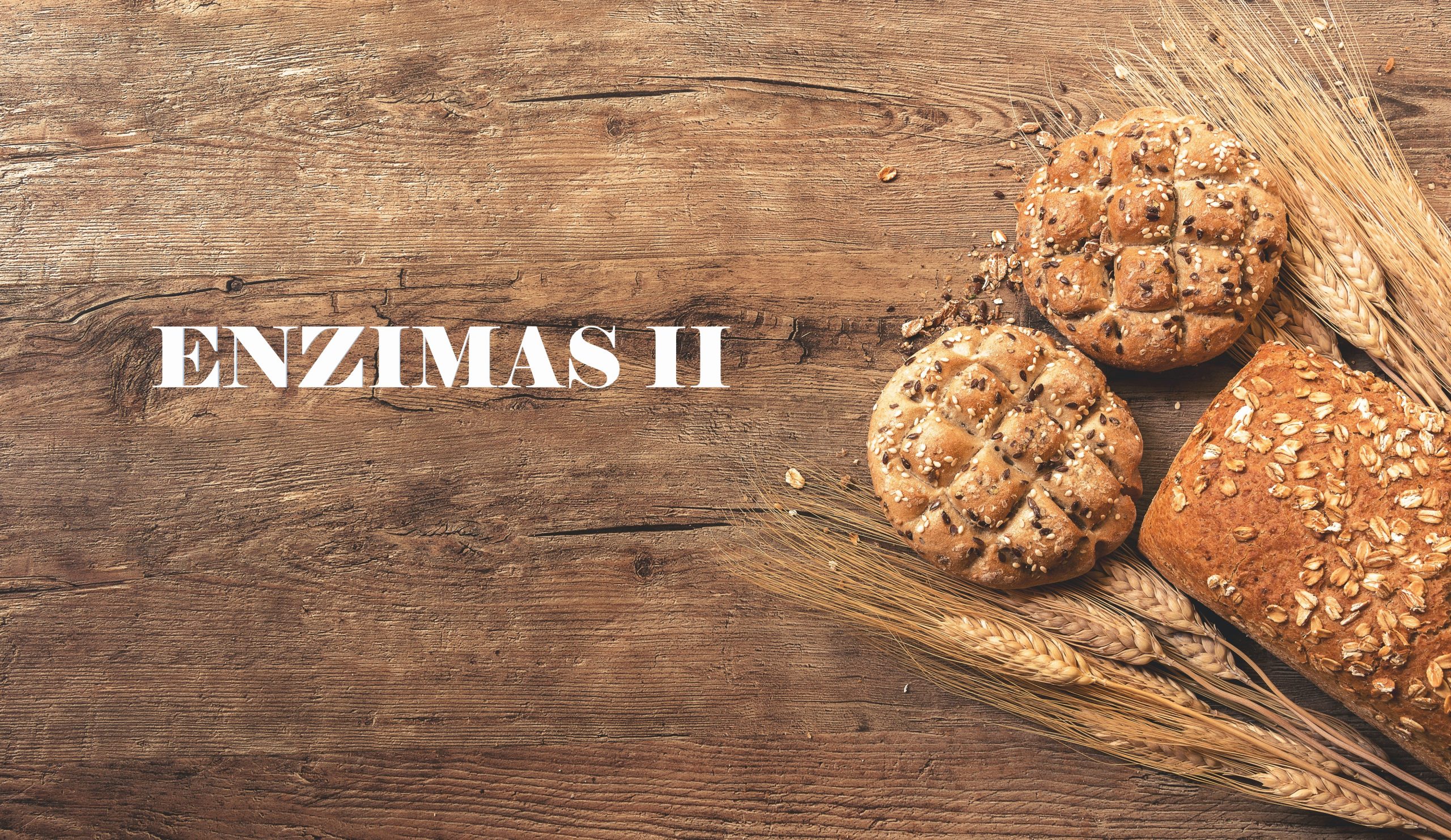
Enzymes II
Today we continue our discussion of enzymes, but this time we will explore some lesser-known enzymes, some of which have recently found applications in baking. You can refer to our previous blog post on enzymes in baking, where we covered general aspects and amylases, in the following link.
And don’t forget that you can subscribe to the blog (on the right side) to receive notifications every time we publish a new post.
Hemicellulases
Hemicellulases are now a common component in most bread improvers. It’s important to note that these enzymes can go by various names, such as pentosanases or xylanases. Hemicelluloses are non-digestible polysaccharides composed of more than one type of monomer. In the case of flours, these are usually xylose, arabinose, and galactose. These monomers are linked by β-1,4 bonds, which are challenging to hydrolyse in the intestinal tract. Nutritionally, they are considered dietary fibres. Since the monomers forming hemicelluloses in wheat flour are pentoses, they are sometimes referred to as pentosans, which is why the enzymes capable of hydrolysing them are called pentosanases. Additionally, the most common pentosans in bread dough are arabinoxylans, which consist of a chain of xyloses with added arabinose branches. Enzymes that can break the xylose chain are known as xylanases, making them a specific type of hemicellulase or pentosanase.
Wheat flour contains a small percentage of hemicelluloses, around 4%. These compounds can be either soluble or insoluble and have a high water-absorbing capacity. In fact, they can absorb nearly a quarter of the water in the dough. Enzymes that hydrolyse these compounds reduce their water-absorbing capacity, releasing water that becomes available to hydrate other components of the dough, such as starch and proteins. When these enzymes are added, the dough becomes less consistent and tenacious, and more extensible, improving its workability, or its ability to be rolled out and shaped. The enhanced extensibility leads to better dough expansion during fermentation and in the early stages of baking. Moreover, these enzymes do not appear to have negative effects on the dough.
In dough made with high percentages of rye flour, the use of these enzymes is particularly important because rye flour contains more arabinoxylans than wheat flour. In such cases, these enzymes are essential for a proper baking process.
Lipases
Although the lipid content in dough is low, lipases, enzymes capable of hydrolysing lipids, can be beneficial in the baking process. Lipids are composed of triglycerides, which, in turn, consist of glycerol and three fatty acids. Lipases work by cleaving these fatty acids. Cutting one of them generates a diglyceride, and cutting two produces a monoglyceride. Most lipases cut the two outermost fatty acids, leaving the middle one intact, thereby producing monoglycerides and free fatty acids. As discussed in the section on emulsifiers, monoglycerides are substances that effectively reduce starch retrogradation and slow down bread staling. Thus, the use of lipases can extend the shelf life of bread without the need for additives, reducing the presence of additives both in the labelling and in the final product.
While early lipases function as described, a new generation of lipases naturally produces products with a similar function to DATEM, an emulsifier that interacts with gluten to enhance dough. These lipases belong to the group of phospholipases, which hydrolyse phospholipids. Like their predecessors, these newer lipases reduce the need for emulsifiers and, consequently, additives. However, while the first group has an anti-hardening effect, the latter enhances dough strength.
The fatty acids generated by lipases can also play a role in the aroma and flavour of the products. In fact, this flavour effect is the basis of their use in cheese production.
Proteases
Proteases are enzymes that hydrolyse proteins. In the context of bread dough, they act by breaking down gluten, reducing its strength. While proteases are not commonly used in bread baking in Spain, they find applications in other countries where stronger flours are used. Proteases can help reduce dough mixing times. They also make the dough more flowable for expansion within a mold and can be useful in other preparations. Overall, the use of proteases has a similar effect to that of reducing agents, which relax the dough by breaking disulfide bonds. However, there are two significant differences. First, the action of reducing agents is reversible, whereas protease-induced protein breakdown is irreversible. Second, while reducing agents have a quick effect at the beginning of mixing, proteases work more slowly, depending on the time and temperature of the dough.
To shorten mixing times effectively, proteases should be added during sponge fermentation, which is then mixed with the rest of the flour. This allows the proteases sufficient time to act, but they do not affect the entire dough. Proteases are also being explored for use in products that need to expand within molds, such as hamburger or hot dog buns, as they make the gluten network less elastic, facilitating lateral expansion. However, the action of proteases on gluten during fermentation can impair its gas-retention capacity, resulting in dough collapse during fermentation or at the beginning of baking.
Proteases can also be useful in making saltine crackers or pizza dough, as they reduce dough elasticity and increase its extensibility. However, excessive proteolysis can be harmful.
A new application of proteases involves improving crusts. Hydrolysing proteins in the crust results in more free amino acids and enhances Maillard reactions, producing darker crusts. What’s less known is that the hydrolysis of proteins in the crust also helps create crisper crusts. If this effect is desired, the protease should be applied to the dough’s surface, not incorporated into the formulation, to avoid degrading the gluten network responsible for gas retention. This application can be valuable in crusty breads production but not in sandwich bread, where the crust should not be crisp.
Among proteases, those acting inside proteins, breaking bonds between amino acids randomly (endo-), are preferred over those working at the ends, cleaving bonds one by one (exo-). The latter have a much smaller effect on dough strength, but they can be used in biscuit production. Additionally, there are different proteases based on their optimal pH and specificity in breaking specific amino acid bonds. Therefore, it’s crucial to choose the right type of protease as not all of them work the same.
Glucose Oxidase
Glucose oxidase is classified as a dough strengthener enzyme. Its function involves converting glucose into gluconic acid and hydrogen peroxide. This enzyme has been used in the production of powdered eggs to reduce glucose content, preventing it from darkening products through Maillard reactions with amino acids from egg proteins. In bread dough, what’s primarily interesting is the generated hydrogen peroxide, a powerful oxidizer. This action strengthens the dough by promoting the formation of disulfide bonds in the gluten network. Therefore, the use of glucose oxidase can partially or entirely replace additives like ascorbic acid. Initially, it was thought that the low glucose levels in dough could limit the effect, and that it might be necessary to increase glucose levels using glucoamylases. However, it was found that the amount of glucose produced by the amylases present in the flour is sufficiently significant.
Transglutaminase
Transglutaminase is an enzyme capable of binding lysine and glutamine, both amino acids found in proteins. This enzyme has been widely used in meat products and fish derivatives to bind these proteins and create meat-like products (restructured meats) from leftover meat scraps adhering to bones. Transglutaminase has also been used as a coadjutant in the production of surimi (seafood analogues made from more economical raw materials). In the case of bread, the low lysine content and the initial high cost of the enzyme delayed its inclusion in dough formulations. Nevertheless, early research showed that the lysine present in wheat proteins is sufficient for transglutaminase to have a pronounced effect.
By binding proteins, transglutaminase has a clear strengthening effect on dough. However, this effect results in dough that is very tenacious, less extensible, and quite dry and challenging to work with. For this reason, transglutaminase has not been widely incorporated into bread improvers. It is possible that, with proper dosing or when combined with other additives or enzymes, it might have a positive impact, but more convenient alternatives exist.
Transglutaminase has also been tested for binding proteins in the production of gluten-free products, but it is incapable of forming a protein network similar to gluten, so it usually is not used in these types of preparations.
Lipoxygenase
Traditionally, soybean flour, more common in North America, or broad bean flour, more prevalent in Europe, has been used as an ingredient in baking. This practice is due to the presence of lipoxygenase in these flours. Lipoxygenase is an enzyme capable of oxidizing specific fatty acids, such as linoleic or linolenic acid, as well as carotenoids and chlorophylls, which are colouring substances. Some lipoxygenases have a more pronounced bleaching effect as they oxidize colouring substances, while others have a more significant dough-strengthening effect by oxidizing fatty acids.
The bleaching effect of lipoxygenases can be a drawback when aiming for a more yellowish colour, such as in pasta production. In fact, for pasta, it is preferable to use durum wheat varieties with low lipoxygenase content and avoid air incorporation during mixing to reduce the effect of lipoxygenases. However, this effect is beneficial when desiring whiter colours. In English-speaking countries, a whitish colour is an important quality factor for bread, making this enzyme highly useful. In Spain, this parameter is less important, and we are accustomed to slightly creamy bread crumb colour. It is more valued in sandwich bread, where this ingredient has traditionally been used.
The oxidation of fatty acids produces substances that enhance tolerance to excessive mixing and strengthen dough by improving the retention of gases generated during fermentation. This effect is related to the enhancement of disulfide bonds in the gluten network but is still not fully explained. For this effect to occur, the presence of oxygen and fatty acids is necessary. Therefore, it happens during mixing, where air is incorporated into the dough, and in the early stages of fermentation. The action of these enzymes is more potent in bread formulations containing a small proportion of oil. For this reason, its use is more frequent in sandwich bread and similar products.
Commercial lipoxygenases are now available, eliminating the need for soy or broad bean flour. It appears that the effect of these enzymes is more dough-strengthening than bleaching. It is also important to note that the oxidation of fatty acids can generate rancid flavours. This effect is more significant in bread with lean formulations but is less noticeable in products containing flavour-rich ingredients like milk, sugar, seeds, or whole-grain flours.
Asparaginase
Asparaginase is a recently introduced enzyme in baking. This enzyme is capable of hydrolysing asparagine, an amino acid present in proteins, into aspartic acid and ammonia. The interest in using asparaginase in baking lies in the fact that this reaction can significantly reduce acrylamide levels. Acrylamide is a compound produced in the Maillard reaction and has been shown to be carcinogenic. Concerns about acrylamide levels in food arose in 2002 after a study by Swedish researchers. Acrylamide is primarily produced through the reaction between asparagine and reducing sugars under conditions of heat and low humidity, such as those found in bread crust during baking. Therefore, the hydrolysis of asparagine by asparaginase can reduce acrylamide levels in the final product.
More Information
- Butt, M.S., Tahir-Nadeem, M., Ahmad, Z., Sultan, M.T. (2008) Xylanases and their applications in baking industry. Food Technology and Biotechnology, 46:22-31.
- Courtin, C.M., Delcour, J.A. (2002) Arabinoxylans and endoxylanases in wheat flour bread-making. Journal of Cereal Science, 35:225-243.
- Gerits, L.R., Pareyt, B., Decamps, K., Delcour, J.A. (2014) Lipases and their functionality in the production of wheat-based food systems. Comprehensive Reviews in Food Science and Food Safety, 13:978-989.
- Joye, I.J., Lagrain, B., Delcour, J.A. (2009) Use of chemical redox agents and exogenous enzymes to modify the protein network during breadmaking – A review. Journal of Cereal Science, 50:11-21.
- Rosell, C.M., Dura, A. (2016) Enzymes in Bakeries. En Chandrasekaran, M (Ed) Enzymes in food and beverage processing. CRC Press-Taylor & Francis Group. Boca Raton, FL (USA)
- Stauffer, C.E. (1990) Functional additives for bakery foods. Van Nostrand Reinhold. New York (USA)